The Daya Bay Reactor Neutrino Experiment Sees Evidence that Electron Neutrinos Turn into Muon Neutrinos
Surprisingly large effect greatly increases the probability that new neutrino experiments will be able to see the differences between matter and antimatter.
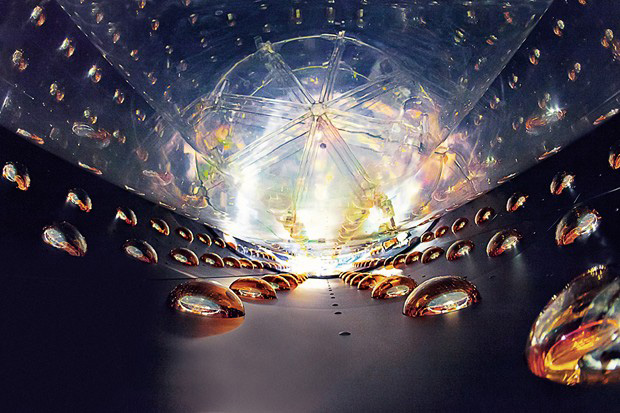
The Science
The Daya Bay Reactor Neutrino Experiment, a multinational collaboration that operates in the south of China, announced on March 8, 2012, its measurement of the disappearance of electron antineutrinos emitted by nuclear reactors operated by China Guangdong Nuclear Power Group. U.S. and Chinese physicists built six underground detectors buried in the mountains near the nuclear reactors. This was the first time that the disappearance of reactor neutrinos has been measured.
The Impact
Neutrinos were first observed to transform between different types in the 1990s when the SuperK experiment observed the disappearance of muon neutrinos due to cosmic rays striking the earth’s atmosphere. This effect was explained by a theory of neutrino oscillations that required that neutrinos have small but non-zero masses. Follow up experiments have all been consistent with that theory, but type of predicted transformation had not been found. This measurement demonstrates the last transformation does occur and provides strong evidence that the theory is correct. Measurement of the strength of oscillations in neutrinos and their antiparticles can be used to search for the cause of the matter and antimatter asymmetry in the universe.
Summary
Physicists have known for years that neutrinos come in three flavors: electron neutrinos, muon neutrons, and tau neutrinos. In 2001 it was experimentally verified that these same neutrinos oscillate into each other, i.e., an electron neutrino can oscillate, or turn into, a muon neutrino. That they do so implies that there is a material substrate that allows these oscillations to occur, i.e., that there are three matter states (physicists call them mass eigenstates) that combine in systematic ways to make up the flavor states that we observe. Measuring the strength of the mixing of these mass eigenstates has been a key to unraveling the mystery of neutrino physics. It is the strength of the mixing of mass eigenstates 1 and 3—represented by a parameter called “theta-1-3”, or Θ13—that was most imprecisely known until Daya Bay announced its results. Knowledge of Θ13, along with the known values of Θ12 and Θ23, refined our ability to calculate the rates at which these mass eigenstates mix to make the various neutrino flavors that we observe. But because the measured value of Θ13 is so surprisingly large, it opens up hope that neutrino experiments on the horizon could be able to observe something known as charge/parity (CP) violation. CP violation has long been observed where quarks are involved, at least in some interactions, but not with leptons (neutrinos are leptons). If CP violation is discovered to also affect neutrino oscillation rates, physicists may then gain insight into one of the fundamental questions of our time: where has all the anti-matter gone?
Contact
Kam-Biu Luk
UC Berkeley
k_luk@lbl.gov
Funding
Basic research: Office of Science High Energy Physics program
Publications
Observation of electron-antineutrino disappearance at Daya Bay, Daya Bay Collaboration (F. P. An et al.), Phys. Rev. Lett. 108 (2012) 171803
Related Links
First Results from the Daya Bay Experiment
Highlight Categories
Performer: University , DOE Laboratory
Additional: Collaborations , International Collaboration