Auroras on the Moon? Which Moon?
Charged particles emanating from Jupiter’s magnetosphere are powered up to create the northern and southern lights on Ganymede, Jupiter’s largest moon.
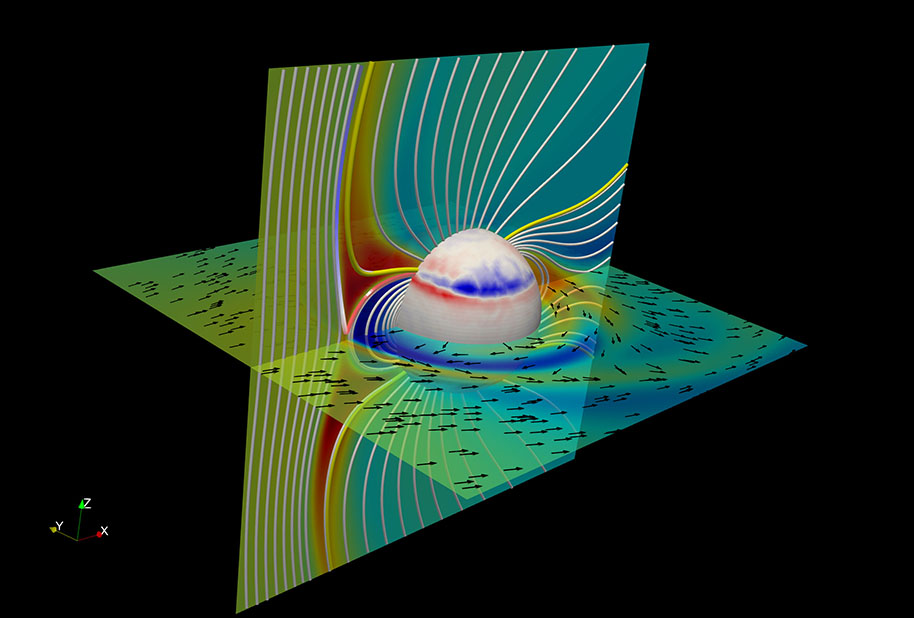
The Science
Ganymede, Jupiter’s largest moon, has a magnetic field much like that of the Earth’s, with magnetic field lines running from north to south. Ganymede, however, is much smaller than Earth, and its field is much weaker. This weak field lets charged particles leak from Jupiter’s atmosphere. The particles are explosively accelerated when the magnetic field lines break apart and then snap back together. Like releasing a slingshot, this ejects very fast electron “projectiles” that hit Ganymede’s surface and excite the bright northern and southern lights that the Hubble Space Telescope has observed. Using advanced supercomputer simulations that cover the moon’s entire magnetosphere, researchers reveal how electrons from Jupiter’s atmosphere are accelerated and collide with the Ganymede polar regions.
The Impact
Ganymede is the only moon in the solar system that displays auroras. Auroras on Earth are beautiful and yield important information about “space weather”—the interaction of the charged particles streaming from the sun with the Earth’s magnetic field. Ganymede’s auroras are simpler than Earth’s, making it easier for scientists to rigorously test their theories. Comparing simulations with observations produces crucial clues about how auroras form on Earth and other planets such as Mercury. The research also helps researchers understand if solar flares will knock out satellites. Also, water affects surfaces that can stabilize aurora formation. By studying Ganymede’s auroras, we can learn if the moon has oceans and could support life.
Summary
High-energy electrons play an important role in auroras. However, previous dynamical simulations either did not incorporate critical electron kinetics or were not capable of studying the entire atmosphere of Ganymede, and important “global” effects were lost. Advanced supercomputer simulations using the Gkeyll code, which was originally developed for fusion energy research at the Princeton Plasma Physics Laboratory, cover the moon’s entire magnetosphere and reveal how electrons from Jupiter’s atmosphere are accelerated and collide with the Ganymede polar regions. These electrons can be powered up to very high energies before hitting the moon’s surface at high latitude, and this greatly affects the strength and location of the bright auroras. The numerical simulations include oxygen ions and electrons, and self-consistently compute their density, momentum, and pressure. It’s particularly important to accurately distinguish between the parallel pressure along the magnetic field and the perpendicular pressure, and between the shear and stress forces where the magnetic field is weak and bends and twists. The model correctly captured key features of Ganymede’s magnetosphere, such as the wing-like structure (called Alfvén wings, shown in figure) and the variations in the brightness of Ganymede’s surface (deducible from the oxygen ions and electrons pressure, shown in figure). The in situ observations from the Galileo spacecraft and remote observations from the Hubble Space Telescope were accurately reproduced.
This work confirms and furthers our understanding of electron physics in magnetospheric dynamics. It sheds light on how the Earth’s magnetic field prevents the solar wind from burning the Earth’s atmosphere to a crisp and the future of predictive technologies for space weather.
Contact
Liang Wang
Princeton University
lw6@princeton.edu
Ammar Hakim
Princeton Plasma Physics Laboratory
ahakim@pppl.gov
Funding
This work was supported by the Department of Energy (DOE) Office of Science’s Office of Fusion Energy Sciences, the National Aeronautics and Space Administration including the “Living With a Star” Jack Eddy Postdoctoral Fellowship Program administered by the University Corporation for Atmospheric Research, Max-Planck/Princeton Center for Plasma Physics, Princeton Plasma Physics Laboratory, Space Science Center of the University of New Hampshire, and the National Science Foundation (NSF). Researchers performed computations on Trillian, a Cray XE6m-200 supercomputer at the University of New Hampshire supported by the NSF Major Research Instrumentation program and the National Energy Research Scientific Computing Center, a DOE Office of Science user facility.
Publications
L. Wang, K. Germaschewski, A. Hakim, C. Dong, J. Raeder, and A. Bhattacharjee, “Electron physics in 3-D two-fluid 10-moment modeling of Ganymede’s magnetosphere.” Journal of Geophysical Research: Space Physics 123, 2815 (2018). [DOI: 10.1002/2017JA024761]
J. Ng, A. Hakim, A. Bhattacharjee, A. Stanier, and W. Daughton, “Simulations of anti-parallel reconnection using a nonlocal heat flux closure.” Physics of Plasmas 24, 082112 (2017). [DOI: 10.1063/1.4993195]
L. Wang, A.H. Hakim, A. Bhattacharjee, and K. Germaschewski, “Comparison of multi-fluid moment models with particle-in-cell simulations of collisionless magnetic reconnection.” Physics of Plasmas 22, 012108 (2015). [DOI: 10.1063/1.4906063]
Highlight Categories
Performer: University , DOE Laboratory , SC User Facilities , ASCR User Facilities , NERSC