Understanding How Semiconductors Absorb Light
Advances in how we calculate optical properties of semiconductors shorten the path to improved solar cells and other optoelectronic devices.
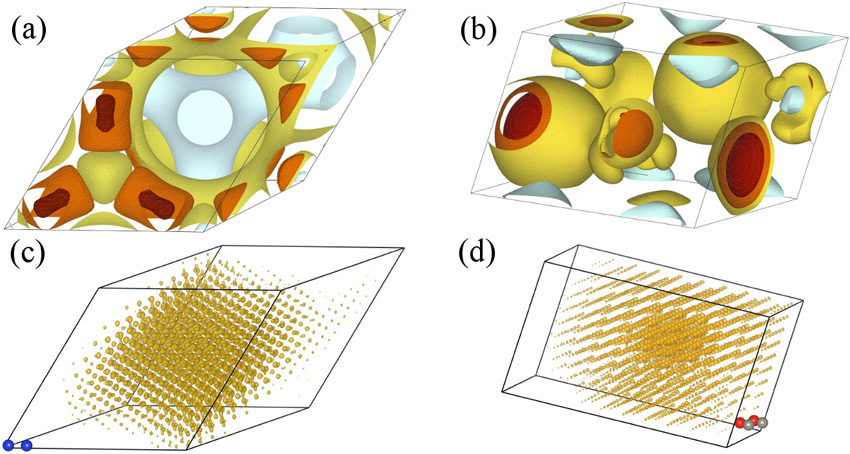
The Science
An advance in the theory describing the absorption of light by semiconductors makes it possible to accurately calculate the properties of composite particles called excitons that significantly affect the performance of solar cells and other optoelectronic devices.
The Impact
Solar-cell performance could be greatly improved by the development of new semiconductor alloys. The ability to calculate optical properties in these materials could lead to large-scale screening via computer calculations – significantly enhancing the rate of discovery as compared to screening by experiment.
Summary
In semiconductors, light absorption creates energetic electrons and electron vacancies or holes, which can roam freely and in solar cells generate an electric current. In some cases, an electron recombines with a hole to create an exciton – a charge-neutral state with a spatial extent many times the atomic spacing. Because exciton formation reduces the photocurrent, understanding the size and binding energy of the exciton is important for optimizing the cell’s efficiency. Standard theory is not accurate enough to predict exciton properties because they depend on the electrical attraction between a negatively charged electron and a positively charged hole, as well as on the complex reorganization of the surrounding electrons in response to the charge. The new approach from the Colorado School of Mines in collaboration with the National Renewable Energy Laboratory, which combines a “many-electron” treatment with a description of the spatial extent of the exciton, provides a shorter route to the optimization of performance than experiment alone. The theory gives excellent agreement with experiment for known semiconductors such as silicon, gallium arsenide, and zinc oxide and provides an explanation for the large binding energy in zinc oxide that had previously puzzled researchers.
Contact
Zhigang Wu
Department of Physics, Colorado School of Mines
Golden, Colorado
zhiwu@mines.edu
Funding
Department of Energy, Office of Science, Basic Energy Sciences Program, through the DOE-SC Early Career Research Program and support of the National Energy Research Scientific Computing Center. Research at the National Renewal Energy Laboratory was supported by DOE’s Office of Energy Efficiency and Renewable Energy.
Publications
M. Dvorak, S.-H. Wei, and Z. Wu. “Origin of the Variation of Exciton Binding Energy in Semiconductors.” Physical Review Letters 110, 016402 (2013). [DOI: 10.1103/PhysRevLett.110.016402]
Related Links
http://prl.aps.org/covers/110/1
Highlight Categories
Performer: University , DOE Laboratory , SC User Facilities , ASCR User Facilities , NERSC
Additional: Collaborations , EERE